Mechanisms of Tolerance Induction
Key insights
Food allergy arises when oral tolerance fails to develop in early life or is breached at an older age. The initial exposure to food allergens occurs predominantly via the gastrointestinal tract or the skin, and can occur at different pre- and postnatal stages. Exposure to food allergens such as peanut and hen’s egg via an inflamed and disrupted epithelial barrier in the absence of oral feeding is an important pathway of allergic IgE sensitization in infants with severe atopic dermatitis. An additional route of allergic sensitization to food could be via the airway tissues.
Current knowledge
Food allergy is defined as an immune-mediated adverse reaction to specific foods. This problem is becoming more widespread and affects up to 8% of children and 5% of adults in Western countries. Currently, there are no effective strategies to induce permanent tolerance: management of food allergies consists of recognizing the adverse reactions and treating the symptoms. Due to the immaturity of their gut barrier and immune system, infants and young children are particularly susceptible to food allergy.
Practical implications
Recent evidence points towards the protective effect of early feeding with peanut and egg in children with eczema, thereby harnessing the pathways that underlie oral tolerance to counteract epicutaneous exposure. An addendum to the NIAID food allergy guidelines recommends the introduction of peanut into the diet of infants with severe eczema or egg allergy, starting at 4–6 months of age. Another approach is to maintain and restore the skin barrier in high-risk infants. Yet another means of inducing gut tolerance is via probiotic and prebiotic supplementation. Despite the promise of oral, epicutaneous, and sublingual methods of food immunotherapy, these have not yet been proven to restore permanent oral tolerance.
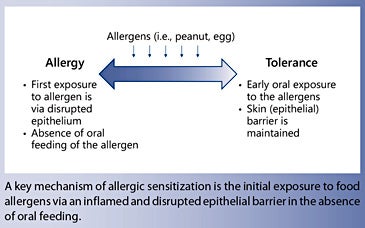
Recommended reading
Von Berg A, Koletzko S, Grubl A, Filipiak-Pittroff B, Wichmann HE, Bauer CP, et al: The effect of hydrolyzed cow’s milk formula for allergy prevention in the first year of life: the German Infant Nutritional Intervention Study, a randomized double-blind trial. J Allergy Clin Immunol 2003;111:533–540.
Du Toit G, Roberts G, Sayre PH, et al; LEAP Study Team: Randomized trial of peanut consumption in infants at risk for peanut allergy. N Engl J Med 2015;372:803–813.
Key Messages
- Early introduction of peanut and egg is associated with a decreased risk of development of allergy to these high-risk foods, especially in infants with severe eczema.
- Restoration of the skin barrier via meticulous and gentle skin care represents another approach to reducing epicutaneous exposure to a food allergen present in the environment and may contribute to a decreased risk of allergic sensitization.
- Interventions aimed at correcting the underlying alterations in the gut microbiota of infants via supplementation with probiotics have potential applications for prevention and treatment of food allergy.
- Oral, epicutaneous, and sublingual immunotherapy induce desensitization in the majority of the treated subjects with food allergy, but their capacity to restore permanent oral tolerance remains unclear.
Key Words
Oral tolerance · Gut-associated lymphoid tissue · Food allergy · Food hypersensitivity · Food allergy prevention · Food allergy treatment · Eczema · Atopic dermatitis · Probiotics · Prebiotics · Microbiota · Desensitization
Abstract
Food allergy results from failure in oral tolerance that usually occurs in infancy or early childhood. Exposure to peanut and hen’s egg via the inflamed and disrupted epithelial barrier in children with severe atopic dermatitis is a risk factor for the development of allergy to these foods and supports the hypothesis that epicutaneous exposure in the absence of oral feeding is an important pathway of allergic IgE sensitization in infants. In recent years, the collective evidence has pointed toward the protective effect of an early feeding with peanut and egg in children with eczema, taking advantage of the pathways underlying oral tolerance to counteract epicutaneous exposure. An addendum to the NIAID food allergy guidelines recommends introduction of peanut into the diet of 4- to 6-month-old infants with severe eczema or egg allergy as an effective strategy to prevent peanut allergy. Strategies aimed at restoring the skin barrier are currently explored as an alternative approach of prevention of eczema and allergic sensitization. Manipulation of the diet via supregplementation with probiotics and prebiotics to restore the healthy gut microbiota represents another potential pathway to induction of tolerance in the gut. Oral, epicutaneous, and sublingual routes of food immunotherapy are promising and induce desensitization in the majority of the treated subjects with food allergy but are not proven to restore permanent oral tolerance. Rigorous multicenter randomized clinical trials are necessary to elucidate the optimal timing, dose, duration, as well as the preventive and therapeutic effects of these diverse approaches.
Introduction
Food allergy is defined as an immune-mediated adverse reaction to food [1]. Food allergy has become an important, global public health problem [2]. Overall, food allergy is estimated to affect up to 8% of children and up to 5% of adults in countries with a so-called Western lifestyle, such as the USA, the UK, Canada, Australia, and Western Europe. The prevalence of peanut allergy documented by a physician-supervised oral food challenge in a population-based cohort of 12-month-old infants in Australia was 3%, reaching epidemic proportions [3]. Currently, there are no proven strategies to induce permanent tolerance; the management relies on recognition of adverse reactions and treatment of symptoms [1, 4]. Considering the risk of fatal anaphylaxis, the negative impact on the nutritional status and quality of life, as well as the cost to the individual and the society, finding effective preventive and therapeutic strategies for food allergy has become a focus of many international research efforts [5, 6].
Food Allergy Risk Factors
Food allergy is most common in infants and young children, as a result of the immaturity of the gut barrier and the immune system in these age groups [7, 8]. Immune deficiencies – including selective IgA deficiency, common variable immunodeficiency, and IPEX (immunodysregulation polyendocrinopathy enteropathy Xlinked syndrome) – are associated with an increased prevalence of food allergy [9]. Genetic factors play an important role in the development of food allergy; however, epigenetic and environmental factors seem to have more relevance in the recent increase in food allergy prevalence (Table 1) [10–18].
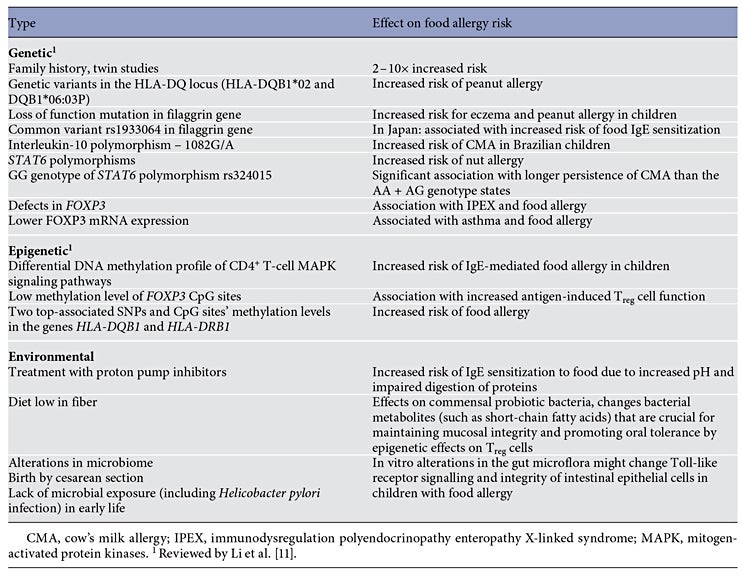
Oral Tolerance
Food allergy results from failure to develop primary oral tolerance or from breach in previously established oral tolerance. Oral tolerance is a state of active nonresponsiveness to ingested soluble antigens mediated by gut-associated intestinal lymphoid tissue. Gut-associated intestinal lymphoid tissue is the largest secondary lymphoid organ in the human body that mounts a protective immune response against a pathogen and ignores a benign antigen, e.g., food or commensal bacteria. Oral tolerance is a highly efficient mechanism that fails in only about 4–8% of the humans who develop food allergy. In mouse models of food allergy, it is very difficult to induce allergic sensitization via oral or parenteral immunization to foods such as cow’s milk, egg white, or peanut included in the diet [19]. However, an exposure through the damaged skin (mimicking a skin barrier defect occurring in atopic dermatitis [AD]) is more likely to induce IgE sensitization to ovalbumin in hen’s egg white and peanut proteins [20, 21].
Mechanism of Oral Tolerance
T cells have been identified as the pivotal cells in oral tolerance based on the experiments in animal models where tolerance can be transferred to naive animals through the transfer of regulatory T (Treg) cells (Table 2) [8].
Inducible FOXP3+ CD4+ Treg cells are central to the maintenance of immune homeostasis and tolerance throughout the body, particularly in the gut [22, 23]. Intestinal FOXP3+ Treg cells regulate mucosal immune responses at multiple cellular levels [24, 25]. Foxp3+ -induced Tregcells are required for oral tolerance and their depletion results in defective oral tolerance in mice and food allergy in humans [26]. Natural development of oral tolerance in food-allergic children is associated with increased Foxp3+ T cells. The resolution of cow’s milk allergy (CMA) in children is associated with an increased frequency of peripheral blood CD4+ CD25+ Treg cells after an oral milk challenge and reduced proliferation of milkspecific T cells [27, 28]. Depletion of CD4+ CD25+ Treg cells restores the in vitro proliferative response in milktolerant individuals [27].
Another cell type important for oral tolerance are CD103+ dendritic cells in the murine and human mesenteric lymph nodes that express high levels of the enzyme retinal dehydrogenase 2 (RALDH2), which converts retinal to retinoic acid. Retinoic acid derived from CD103+ dendritic cells determines gut-homing activity and regplementation ulatory activity of responder T cells. CD103+ dendritic cells also promote the development of Treg cells from naive T cells as well as via indoleamine 2,3-dioxygenase and secretion of transforming growth factor β (TGF-β) [29–32].
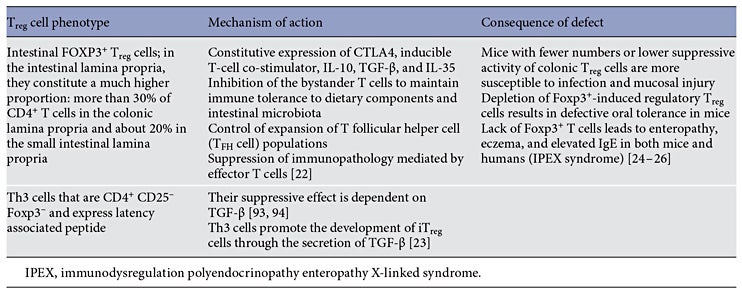
Allergic Sensitization
Food allergy develops when oral tolerance fails to develop early in life or is breached at an older age. Allergic sensitization refers to the event when, following an initial exposure to an antigen, presentation by antigen-presenting cells leads to antigen-specific immune reactions, including the generation of antigen-specific T lymphocytes and the production of antigen-specific IgE antibodies by plasma cells and subsequent binding to its high-affinity receptors on the surface on mast cells and basophils. The initial exposure to food allergens occurs predominantly via the gastrointestinal tract or the skin. The initial contact and subsequent sensitization with a food allergen can occur at different stages of pre- and postnatal life.
The initial exposure to allergens may occur prenatally [33–35]. The immunologic environment of the placenta likely plays a critical role in the development of the fetal immune system. A recent study examining the influence of in vitro allergen exposure in human placentae showed a distinct cytokine/chemokine milieu in allergic and nonatopic mothers with increased allergen-induced placental IL-6 and TNF-α production in atopic mothers. This might explain the higher incidence of sensitization in off-spring of allergic mothers [36–38]. However, modification of the maternal diet during pregnancy did not influence the development of food allergies in the infant later in life seen in the analysis of multiple interventional studies following children up to 10 years of age [39].
Presence of food allergens in breast milk might trigger sensitization in the infant [40, 41]. For many years, guidelines have recommended avoidance of peanut and tree nuts for the first 3 years of life and some also included avoidance during pregnancy (AAP Committee on Nutrition, 2000). These recommendations have been modified after studies failed to show a correlation between maternal diet and development of atopic disease [42]. In contrast to past recommendations, more recent studies have shown a protective effect of high allergen consumption during pregnancy [43]. High consumption of peanuts and tree nuts during pregnancy was found to be associated with lower rates of food allergy in children [44]. A study investigating associations between maternal consumption of common childhood allergens during pregnancy and childhood outcome of allergic disease and asthma showed a decreased incidence of asthma and atopic disease at the age of 8 years in children whose mothers had a high consumption of peanut, milk, and wheat during early pregnancy [45]. It is likely that other routes of sensitization like transcutaneous exposure are key factors in the development of food allergy.
A clear association has been shown between early onset of AD and the development of food allergies [46]. The impaired skin barrier leads to increased transcutaneous passage of antigens and subsequent sensitization. Children with severe AD who used skin care products containing peanut oil showed higher rates of peanut sensitization, supporting the theory of transcutaneous sensitization [46]. It was found that about 50% of children with moderate-to-severe AD had a loss of function mutation of filaggrin and also showed increased sensitization to peanut [10]. This was also noted in mouse models: filaggrin-deficient mice showed a Th17-dominated skin inflammation and susceptibility to epicutaneous sensitization [47]. In mouse models of egg and peanut allergy, skin exposure to these foods promotes the development of specific IgE sensitization to ovalbumin and peanut, whereas an oral exposure promotes oral tolerance [20, 21]. Cutaneous exposure to food antigens induces thymic stromal lymphopoietin production, activation of basophils that produce IL-4, production of Th2 cytokines, and accumulation of mast cells in the gut [52]. Mutations in genes encoding proteins that determine the integrity of the skin barrier, such as FLG encoding filaggrin, are independent risk factors for peanut allergy [52]. Figure 1 describes differential immune responses to food protein in the gastrointestinal tract and skin.
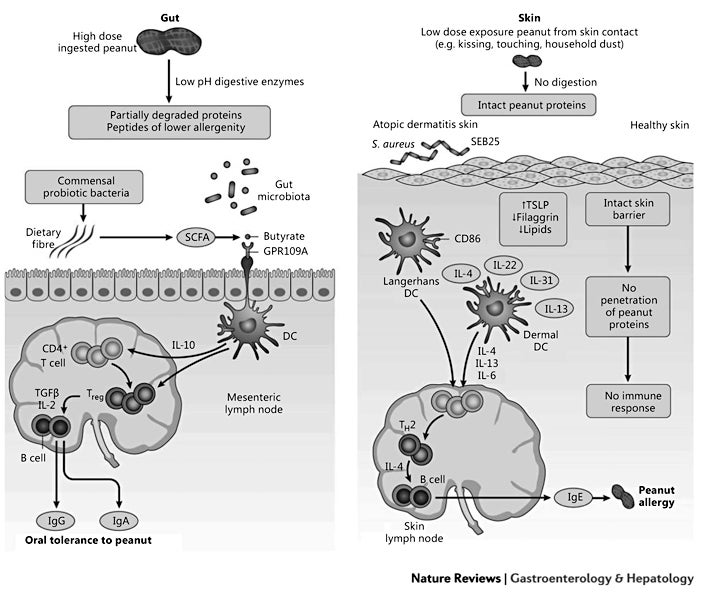
Sensitization via Food Ingestion
The introduction of food proteins as in infant formula or complementary food leads to a change in the infant’s gut microbiota, and the relative immaturity of the infant’s digestive tract may allow for passage of allergens in a form or amount that will trigger allergic sensitization rather than tolerance (Table 3).
An additional route of allergic sensitization to food could be via the airways, as seen in the occupational settings (e.g., food industry) with exposure to aerosolized food proteins such as wheat and egg [53]. Additionally, systemic reactions to ingested egg can occur in adults exposed to pet bird dander via inhalation due to the presence of a cross-reactive antigen, alpha-livetin in both the dander and egg yolk [54]. It remains to be determined whether primary sensitization via the airways might occur in infants with gastroesophageal reflux through microaspiration of the gastric content.
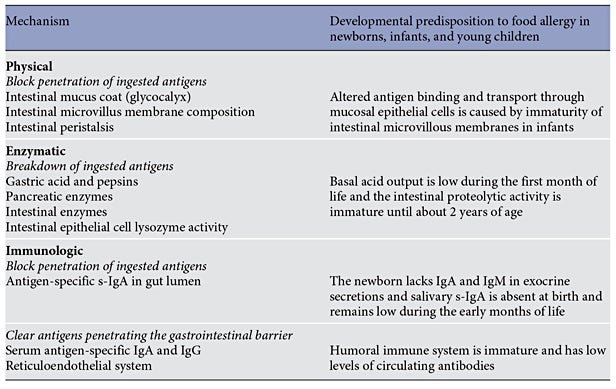
Tolerance Induction for Food Allergy Prevention
There is no consensus whether food allergies can be prevented and what is the optimal duration of exclusive breastfeeding as well as timing of supplemental formula and solid food introduction.
Breastfeeding
Human breast milk contains variety of bioactive molecules which are involved in infant growth, actively modulate immune system and gut microbiota, confer passive immunity, and have a positive impact on cognitive development and metabolism [55]. Breast milk contains gut trophic factors (epidermal growth factor, insulin growth factor, and TGF-β), capable of actively stimulating crypt and villi formation, decreasing intestinal permeability in the first week of life. In addition, lactoferrin and vitamin A in breast milk may affect the neonatal gut barrier. Human milk oligosaccharides downregulate CD14 expression in human enterocytes, and epidermal growth factor suppresses TLR4 signaling, leading to attenuation of lipopolysaccharide- induced inflammation [56]. Breast milk is thought to contribute to the diversification of the neonatal microbiota via maternal IgA. Several studies have provided evidence for breast milk to contain bacteria, approximately 103–104 CFU/mL, suggesting that breast milk microbiome may provide a source of commensal bacteria for the infant gut.
Food protein transfer via breast milk is the first exposure to foods for the infant. In a mouse model, mice exposed to nanograms of egg ovalbumin antigen through breast milk were protected from ovalbumin-induced allergic airway disease and TGF-β from breast milk was critical for tolerance induction, suggesting that variability in breast milk allergen content, TGF-β, and allergen-specific immunoglobulin may contribute to heterogeneity of results on allergy prevention by breastfeeding. Presence of peanut in maternal diet was associated with a reduced risk of food allergy in the offspring in both humans and mice [41, 45, 57]. Collectively, there is currently no conclusive evidence that breastfeeding protects from development of food allergy, potentially reflecting the environmental factors that affect the composition of maternal milk. It remains to be determined whether maternal supplementation with probiotic and/or vitamin A might improve the pro-tolerogenic capacities of human breast milk [58, 59]. Currently, the general consensus is that breastfeeding for at least 6 months should be promoted in view of the known and recognized nutritional and immunological benefits of breast milk [60].
Infant Formula
Considering that intact food proteins have the highest allergenicity and the efficacy of hypoallergenic infant formulas in the dietary management of established CMA, a variety of hydrolyzed formulas based on cow’s milk protein (CMP) have been investigated for the prevention of food allergy and atopic diseases. It has been hypothesized that partially hydrolyzed proteins (whey- or casein-derived peptides of various molecular weights) will result in better oral tolerance induction in a setting of immature gastrointestinal and immune systems, compared to intact CMP. The largest and most rigorous clinical trial to investigate the preventative effect of hypoallergenic formulas, the German Infant Nutritional Intervention Study (GINI Study) reported that infants fed extensively hydrolyzed casein formula (EHCF) for the first 4 months had a reduced rate of AD at 1 year of age compared with infants fed cow’s milk formula (CMF) [61]. Feeding with partially hydrolyzed whey or or EHCF was associated with a reduced rate of AD but not of asthma or allergic rhinitis at the ages of 3, 6, 10, and 15 years compared with feeding with CMF [62–65]. However, a meta-analysis of 37 eligible intervention trials of hydrolyzed formula including over 19,000 participants concluded that there was no consistent evidence that partially or extensively hydrolyzed formulas reduce the risk of allergic outcomes in infants at high risk [66]. A limitation of the meta-analysis is that it compared studies using different hydrolyzed formulas within each category. This approach is questionable because different biological effects of various hydrolysates are not only based on molecular mass distribution, but also on different peptide characteristics and sequence profiles.
A large single-center prospective study from Israel examined the prevalence of CMA in 13,019 infants followed up for 2 years [67]. CMP was introduced to healthy infants at a mean age of 61.6 ± 92.5 days and to infants with IgE-mediated CMA at 116.1 ± 64.9 days. The odds ratio (OR) for developing CMA was 19.3 (95% confidence interval [CI] 6.0–62.1) among infants exposed to CMP after more than 15 days compared with those exposed in the first 14 days of life. These findings suggest that early exposure to CMP may be protective against the development of CMA [67].
Timing of Foreign Food Protein Introduction
It was once assumed that the avoidance of allergenic foods and delayed introduction into the diet would prevent allergy by avoiding a so-called “window of physiologic susceptibility” associated with developmental immaturity of the gastrointestinal and immune systems (Table 3). However, the implementation of these expert opinion-based guidelines has been paralleled by a significant increase in the prevalence of peanut allergy in the societies with a so-called Western lifestyle, such as the USA, the UK, Australia, and Western Europe [2]. Subsequent studies determined that risk of peanut allergy is highest in infants with severe eczema, in those with mutations in filaggrin gene resulting in an impaired skin barrier function, and in those not eating peanut but exposed to peanut in the household dust. In addition, the prevalence of peanut allergy was 10-fold higher among Jewish children in the UK compared with Israeli children of similar ancestry [68]. In Israel, peanut-containing foods are usually introduced in the diet when infants are approximately 7 months old and consumed in substantial amounts, whereas in the UK children do not typically consume any peanut-containing foods during their first year of life [68]. These observations inspired a number of clinical trials investigating the early introduction of solid foods for prevention of food allergy [4].
Peanut
A landmark clinical trial (Learning Early About Peanut Allergy, LEAP) randomized 640 infants between the ages of 4 and 11 months with severe eczema, egg allergy, or both (considered at high risk for peanut allergy) to consume or avoid peanut until 60 months of age ( Table 4 ) [48]. Early introduction of peanut dramatically (overall by 81%) decreased the development of peanut allergy among children at high risk for this allergy. Early oral introduction of peanut induces oral tolerance that precedes potential IgE sensitization to peanut via the disrupted skin barrier. Considering the strong protective effect of this intervention and the size of the clinical trial, an addendum to the 2010 NIAID guidelines for food allergy diagnosis and management has been published in 2017 [69]. The guidelines recommend introducing peanutcontaining foods in age-appropriate forms to infants at risk (with severe eczema, egg allergy, or both) preferably during breastfeeding, starting at the age of 4–6 months. The document provides practical recommendations on the safe introduction of peanut to such infants. In addition, the guidelines recommend introducing peanut to infants with mild-to-moderate eczema around 6 months of age. For infants without eczema or any food allergy, free introduction of peanut into the diet, together with other solid foods that are age-appropriate, as per family preferences is recommended.
High-Risk Foods (Peanut, Cooked Egg, Cow’s Milk, Wheat, Sesame, and Whitefish)
A similar concept has been tested in the EAT trial that evaluated whether the early introduction of allergenic foods into the diet of breastfed infants would protect against the development of food allergy [49]. The EAT trial recruited 1,303 exclusively breastfed infants from the general population who were 3 months of age. They were randomly assigned to the early introduction of 6 allergenic foods (peanut, cooked egg, cow’s milk, sesame, whitefish, and wheat; early-introduction group) or to the current practice recommended in the UK of exclusive breastfeeding to approximately 6 months of age (standard- introduction group). The primary outcome was food allergy to 1 or more of the 6 foods in children between 1 and 3 years of age (Table 4). The trial did not show the efficacy of early introduction of allergenic foods in an intention-to-treat analysis. Further per-protocol analysis raised the question of whether the prevention of food allergy by means of early introduction of multiple allergenic foods was dose dependent. The EAT trials also demonstrated that the early introduction of solids is not easy and may not be practical for many families.
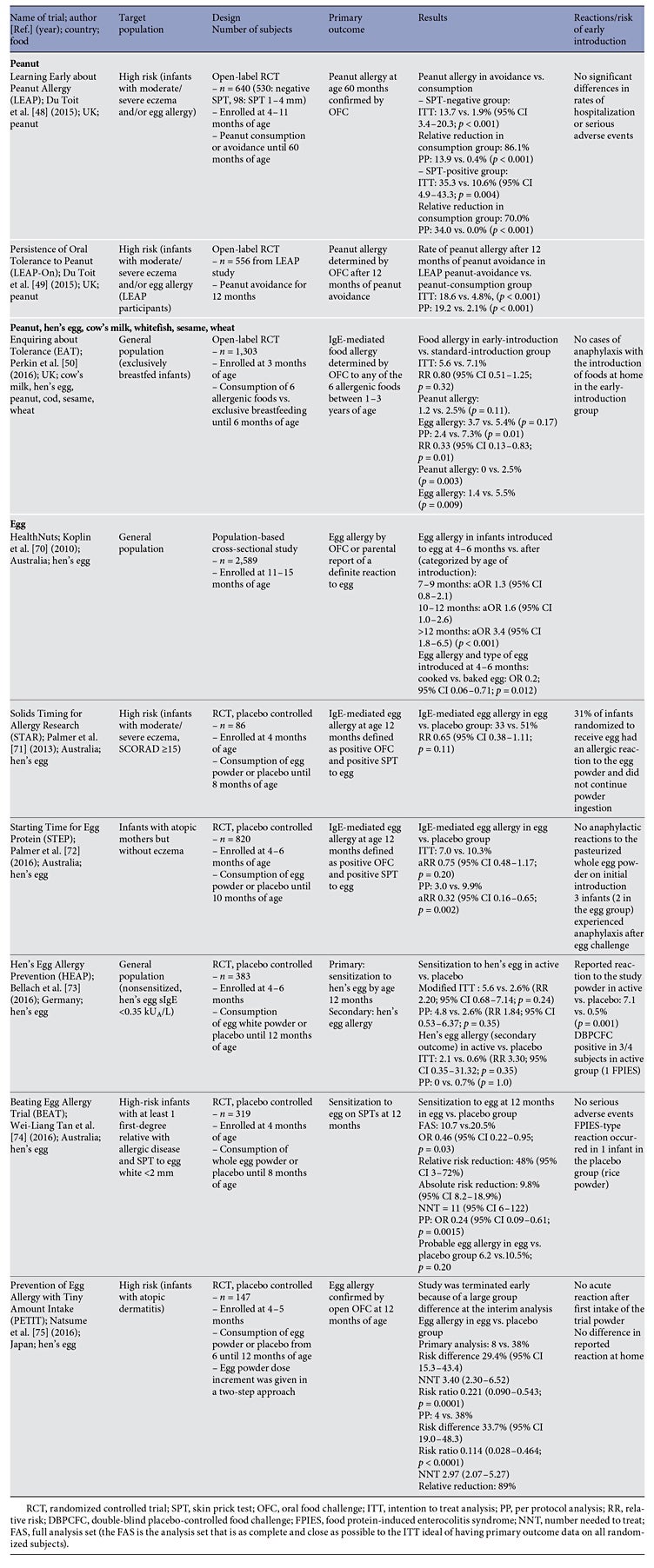
Egg
A number of clinical trials investigated the early introduction of egg (Table 4) [70–75]. The overall effect was that the early introduction of egg may confer a preventative effect, although sensitization to egg occurs early and allergic infants may develop anaphylaxis on a first known ingestion of egg white powder regardless of their eczema status [76] . It is likely that the early introduction of baked or lightly cooked egg is safer.
Potential Pitfalls of Early Food Introduction
While the current cumulative evidence favors the early introduction of highly allergenic foods, there are a number of potential problems that have to be considered. Infants without obvious risk factors such as eczema, other food allergies, or a family history of food allergy may develop food allergy and manifest anaphylaxis on a first known ingestion, in particular of egg. This suggests that an even earlier introduction of egg is necessary but may not be feasible. As demonstrated by the EAT study, early (starting at 3 months) introduction of egg, peanut, sesame, and fish may be challenging because age-appropriate forms of these foods are not easily accessible to families. Regular intake of the food, at least 2–3 times per week, is necessary, and some families struggle with incorporating high-risk foods into the daily diet over prolonged periods of time, especially if the food is not a part of the regular family diet. Finally, the studies focus on prevention of IgE-mediated food allergy, and the effects of early introduction on the development of non-IgE-mediated food allergy is unknown. In the LEAP trial, there was 1 case of peanut food protein-induced enterocolitis syndrome (FPIES). In the EAT trial, there were 10 participants whose families reported FPIES-like reactions (median age, 5 months): 7 in the early-introduction group (6 reporting egg as the trigger, 1 sesame) and 3 in the standard- introduction group (1 fish and prawn, 1 milk, and 1 milk, soya, and rice) (p = 0.34). When challenges were undertaken (median age, 16 months) of the 7 early-introduction group participants, 5 had negative challenges, 1 was positive, and 1 did not return for the challenge. Of the 3 standard-introduction group participants, 2 had positive challenges and 1 had a negative challenge. In the HEAP trial, there was 1 case of egg FPIES in the active group, raising concerns about increasing risk for the development of FPIES with early introduction of allergenic foods.
Strategies to Restore the Healthy Gastrointestinal Microbiota
Probiotics
Probiotics are defined as live bacteria that naturally colonize the gastrointestinal tract, and their presence in adequate amounts is associated with health benefits for the host [77]. An altered composition of the gut microbiota might predispose children to food allergy by changing Toll-like receptor signaling and the integrity of intestinal epithelial cells [15]. In a gnotobiotic mouse model, selective colonization of the gut with Clostridia-containing microbiota protects from food allergy via activation of innate lymphoid cells, IL-22 production, and enhancement of intestinal permeability [16]. The gut microbiota may also play a role in the natural history of CMA. Additional potential mechanisms by which probiotics exert pro-tolerogenic effects in the gut are illustrated in Figure 2.
Among 226 children with milk allergy who were enrolled at infancy in the Consortium of Food Allergy observational study of food allergy, the gut microbiome composition at the age of 3–6 months was associated with milk allergy resolution by the age of 8 years (PERMANOVA, p = 0.047), with enrichment of Clostridia and Firmicutes in the infant gut microbiome of subjects whose milk allergy resolved [78]. Metagenome functional prediction supported decreased fatty acid metabolism in the gut microbiome of subjects whose milk allergy resolved ( η2 = 0.43; ANOVA, p = 0.034). Therefore, early infancy is a window during which the gut microbiota may determine food allergy outcomes in childhood. Bacterial taxa within Clostridia and Firmicutes could be studied as probiotic candidates for milk allergy therapy.
Supplementation with probiotics has been shown to exert anti-inflammatory properties together with cytokine changes that might skew towards Th1-biased responses and inhibit Th2-biased responses and IgE production. Probiotics were shown to increase secretion of IL-10 and TGF-β by upregulating Treg cells (Fig. 2). A meta-analysis of the randomized controlled clinical trials investigating the use of probiotics in infants for primary prevention of allergies found mild reduction in clinical eczema in infants but insufficient evidence for a general recommendation of probiotic supplementation for prevention of allergic disease or food hypersensitivity [79]. It remains to be determined whether supplementation with probiotic bacteria can correct the underlying alterations in the gut microbiota in children with food allergy [18].
Prebiotics
Prebiotics are food components that are nondigestible and reach the colon where they provide nutrition and stimulate growth and activity of bacteria of the normal gut flora (Fig. 2). They are commonly added as nutritional supplements like oligosaccharides [77]. Pooling of data from multiple studies in a recently updated Cochrane review showed a potential benefit in the prevention of AD, but no conclusive evidence was found regarding the prevention of other allergic diseases or food allergies [80]. In a parallel-group, multicenter, randomized double-blind controlled trial of partially hydrolyzed whey formula containing oligosaccharides (pHF-OS) versus standard CMF, infants with a family history of allergic disease were randomized (stratified by center/maternal allergy) to pHFOS (n = 432) or CMF (n = 431) until 6 months of age if the formula was introduced before 18 weeks of age. The primary outcome was cumulative incidence of AD by 12 months in infants randomized at 0–4 weeks (pHF-OS, n = 375; control, n = 383). At 12 months, there was no difference in AD in the infants fed with study formula (07/347; 30.8%) compared to the infants fed with CMF (112/370; 30.3%; OR 0.99, 95% CI 0.71–1.37; p = 0.94). pHF-OS did not change most immune markers including total/specific IgE; however, pHF-OS reduced cow’s milkspecific IgG1 (p < 0.0001) and increased Treg cell and plasmacytoid dendritic cell percentages [81].
Microbiome Restoration in Infants Born via Cesarean Section
Exposure of newborns to the maternal vaginal microbiota is interrupted with cesarean birthing. Babies delivered by cesarean section (C-section) acquire a microbiota that differs from that of vaginally delivered infants, and Csection delivery has been associated with increased risk for immune and metabolic disorders. In a pilot study, infants delivered by C-section were exposed to maternal vaginal fluids at birth [82]. As in vaginally delivered babies, the gut, oral, and skin bacterial communities of these newborns during the first 30 days of life were enriched in vaginal bacteria – which were underrepresented in unexposed C-section- delivered infants – and the microbiome similarity to those of vaginally delivered infants was greater in oral and skin samples than in anal samples. Although the long-term health consequences of restoring the microbiota of C-section- delivered infants remain unclear, these preliminary results demonstrate that vaginal microbes can be partially restored at birth in C-section-delivered babies [82, 83].
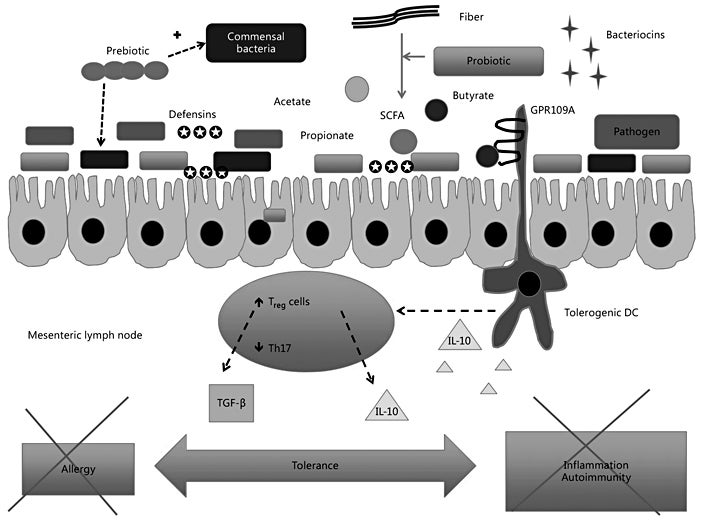
Skin Barrier Restoration
Considering that an impaired and inflamed skin barrier is a hallmark of AD and a risk factor for the development of peanut allergy, strategies aimed at the restoration and protection of the skin barrier represent an alternative approach to prevent food allergy. Two small clinical trials conducted in the USA and Japan reported a reduction in eczema prevalence in infants at risk [84, 85].
In an Irish birth cohort, transepidermal water loss (TEWL) was measured at birth (day 2) and at 2 and 6 months in 1,903 infants [86]. The prevalence of AD was 18.7% at 6 months and 15.53% at 12 months. In a logistic regression model, upper-quartile TEWL measurement on day 2 of life strongly predicted AD at 12 months (area under the receiver operating characteristic curve, 0.81; p < 0.05). Lowest-quartile TEWL on day 2 of life was protective against AD at 12 months. An upper-quartile TEWL at the age of 2 months was also strongly predictive of AD at 12 months (area under the receiver operating characteristic curve, 0.84; p < 0.05). At both ages, this effect was independent of parental atopy, filaggrin status, or report of an itchy flexural rash at 2 months. Associations were increased when the parental atopy status or child filaggrin mutation status were added into the linear regression model. Furthermore, food IgE sensitization was present in 6.27%, and food allergy prevalence was 4.45% [86] . Egg was the most prevalent allergen (2.94%), followed by peanut (1.75%) and cow’s milk (0.74%). Day 2 upper-quartile TEWL (>9 g water/m 2 /h) was a significant predictor of food allergy at the age of 2 years (OR 4.1, 95% CI 1.5–4.8). Day 2 TEWL was in the upper quartile in 75% of children with food allergy at 2 years of age. Even in those without AD, infants with upper-quartile day 2 TEWL were 3.5 times more likely to have food allergy at 2 years than infants in the lowest quartile (95% CI 1.3– 11.1; p = 0.04). This important study demonstrated that an impairment of skin barrier function at birth and at 2 months precedes clinical manifestations of AD. In addition to providing important mechanistic insights into disease pathogenesis, these findings have practical implications for the optimal timing of interventions for the prevention of AD. Neonatal skin barrier dysfunction predicts food allergy at 2 years of age, supporting the concept of transcutaneous allergen sensitization, even in infants who do not have AD. TEWL could be used for stratifying infants in the first few days of life before development of AD or food allergy for targeted intervention studies to potentially alter the atopic march. Currently, large clinical trials are underway to enhance the skin barrier from birth, using emollients and decreasing bathing frequency, to reduce the incidence of AD and food allergy in highrisk neonates.
Tolerance Induction for Food Allergy Treatment
Hypoallergenic Formula with Probiotics in CMA
A nonrandomized study investigated 260 Italian children (median age, 5.92 months) diagnosed with CMA, both IgE-mediated (42.7%) and non-IgE-mediated. Children were fed with: EHCF (n = 55); EHCF + Lactobacillus rhamnosus GG (LGG) (n = 71); hydrolyzed rice formula (n = 46); soy formula (n = 55); and amino acidbased formula (n = 33). The formula choice was at the discretion of the managing physician. The rate of children acquiring oral tolerance after 12 months (determined by an oral food challenge) was significantly higher ( p < 0.05) in the groups receiving EHCF (43.6%) or EHCF + LGG (78.9%) compared with the other groups (hydrolyzed rice formula [32.6%], soy formula [23.6%], and amino acid-based formula [18.2%]). The rate of tolerance acquisition was influenced by 2 factors: (1) IgEmediated mechanism (B –2.05, OR 0.12, 95% CI 0.06– 0.26; p < 0.001); and (2) formula choice, such that those receiving either EHCF (B 1.48, OR 4.41, 95% CI 1.44– 13.48; p = 0.009) or EHCF + LGG (B 3.35, OR 28.62, 95% CI 8.72–93.93; p < 0.001). This study suggested that EHCF (especially with added LGG) may accelerate tolerance acquisition in children with CMA compared to other formulas [88]. Feeding with EHCF + LGG led to a significant increase in fecal butyrate levels, suggesting that EHCF + LGG promotes tolerance in infants with CMA, in part, by influencing the strain-level bacterial community structure of the infant gut [18]. In a follow-up study, 40 children (aged 3–18 months) were enrolled: 10 children with active IgE-mediated CMA (group 1), 10 children who outgrew CMA after dietary treatment with an EHCF containing the probiotic LGG (group 2), 10 children who outgrew CMA after treatment with other formulas (group 3), and 10 healthy controls (group 4). FoxP3 TSDR demethylation and expression were measured in mononuclear cells purified from peripheral blood of the 4 groups of children. FoxP3 TSDR demethylation was significantly lower in children with active IgE-mediated CMA than in either children who outgrew CMA or in healthy children. Formula selection influenced the FoxP3 TSDR demethylation profile, suggesting that tolerance acquisition in children with IgE-mediated CMA involves epigenetic regulation of the FoxP3 gene.
A more rigorous parallel-arm randomized controlled trial investigated whether the administration of EHCF containing the probiotic LGG can reduce the occurrence of other allergic manifestations. Children with IgE-mediated CMA were randomly allocated to the EHCF or EHCF + LGG groups and followed up for 36 months [89]. The main outcome was the occurrence of at least 1 allergic manifestation (eczema, urticaria, asthma and rhinoconjunctivitis) diagnosed according to standardized criteria. Tolerance acquisition was evaluated every 12 months. A total of 220 children (147 males, 67%) with a median (IQR) age of 5.0 (3.0–8.0) months were randomized: 110 children were placed in the EHCF group and 110 children were placed in the EHCF + LGG group. In the complete case analysis, the absolute risk difference for the occurrence of at least 1 allergic manifestation over 36 months was –0.23 (95% CI –0.36 to –0.10; p < 0.001) and the absolute risk difference for the acquisition of cow’s milk tolerance was 0.20 (95% CI 0.05–0.35; p < 0.01) at 12 months, 0.24 (95% CI 0.08–0.41; p < 0.01) at 24 months, and 0.27 (95% CI 0.11–0.43; p < 0.001) at 36 months. This study suggested that EHCF + LGG might reduce the incidence of other allergic manifestations and hasten the development of oral tolerance in children with IgE-mediated CMA. The results are very interesting and should be replicated in different patient populations.
Immunotherapy for Food Allergy
There are no currently approved therapies for food allergy; a number of immunotherapeutic strategies are currently being evaluated for IgE-mediated food allergy [89]. All of them rely on regular exposure to the food allergen via the oral (oral immunotherapy, OIT), sublingual (sublingual immunotherapy), or epicutaneous (epicutaneous immunotherapy) route; subcutaneous vaccines based on modified hypoallergenic major peanut allergens are currently undergoing phase I clinical trials in adults [5, 6]. While OIT induces a temporary state of increased threshold of clinical reactivity to the food allergen, dependent on daily OIT dosing (referred to as desensitization), no food immunotherapy is proven to induce/restore permanent oral tolerance (Table 5). Improvements in safety allowing for the inclusion of patients with severe phenotypes of food allergy and asthma, refinements in dose and duration of treatment to enhance efficacy, and understanding of the mechanisms underlying desensitization and tolerance are desirable. Emerging evidence suggests that early initiation of peanut OIT in infants and young children may offer superior efficacy and safety due to lower OIT doses used and a more favorable response to immunomodulation in a developing immune system [91]. Large clinical trials of rigorous design and adequate sample sizes are necessary to fully evaluate the effects of food immunotherapy. Alternative approaches utilizing a combination of several synergistic treatments (e.g., OIT and anti-IgE monoclonal antibody or OIT and probiotics) or modified hypoallergenic molecules combined with adjuvants may be necessary for patients with the most severe phenotypes of food allergy [92, 93].
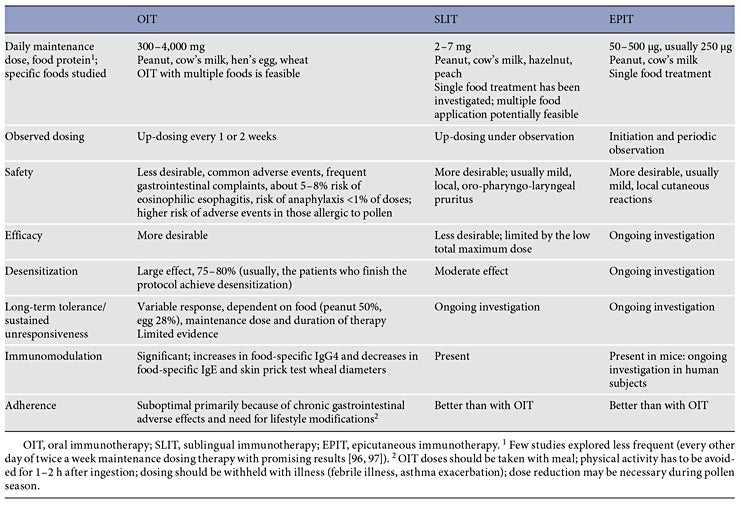
Summary
Food allergy results from failure in oral tolerance that usually occurs in infancy or early childhood. Exposure to food allergen such as peanut and hen’s egg via the inflamed and disrupted epithelial barrier in the absence of oral feeding is an important pathway of allergic IgE sensitization in infants with severe AD. In recent years, the collective evidence has pointed toward the protective effect of an early feeding with peanut and egg in children with eczema, taking advantage of the pathways that underlie oral tolerance to counteract epicutaneous exposure. An addendum to the NIAID food allergy guidelines recommends the introduction of peanut into the diet of infants with severe eczema or egg allergy, starting at 4–6 months of age, as an effective strategy to prevent peanut allergy. Strategies aimed at restoring the skin barrier are currently explored as an alternative approach of prevention of eczema and allergic sensitization. Manipulation of diet via supplementation with probiotics and prebiotics to restore the healthy gut microbiota represents another potential pathway to induction of tolerance in the gut. Oral, epicutaneous, and sublingual routes of food immunotherapy are promising and induce desensitization in the majority of the treated subjects but are not proven to restore permanent oral tolerance. Rigorous multicenter randomized clinical trials are necessary to elucidate the optimal timing, dose, duration, as well as the preventive and therapeutic effects of these diverse approaches.
Disclosure Statement
A.N.-W. is a member of the Gerber Advisory Council and received research funding from Nutricia and Nestlé. P.C. reports no conflict of interest. The writing of this article was supported by Nestlé Nutrition Institute.
References
- Sampson HA, Aceves S, Bock SA, James J, Jones S, Lang D, et al: Food allergy: a practice parameter update – 2014. J Allergy Clin Immunol 2014; 134: 1016–1025 e43.
- Prescott SL, Pawankar R, Allen KJ, Campbell DE, Sinn J, Fiocchi A, et al: A global survey of changing patterns of food allergy burden in children. World Allergy Organ J 2013; 6: 21.
- Osborne NJ, Koplin JJ, Martin PE, Gurrin LC, Lowe AJ, Matheson MC, et al: Prevalence of challenge-proven IgE-mediated food allergy using population-based sampling and predetermined challenge criteria in infants. J Allergy Clin Immunol 2011; 127: 668–676.
- Wood RA: Food allergen immunotherapy: current status and prospects for the future. J Allergy Clin Immunol 2016; 137: 973–982.
- Albin S, Nowak-Wegrzyn A: Potential treatments for food allergy. Immunol Allergy Clin North Am 2015; 35: 77–100.
- Feuille E, Nowak-Wegrzyn A: Oral immunotherapy for food allergies. Ann Nutr Metab 2016; 68(suppl 1):19–31.
- Kalach N, Rocchiccioli F, de Boissieu D, Benhamou PH, Dupont C: Intestinal permeability in children: variation with age and reliability in the diagnosis of cow’s milk allergy. Acta Paediatr 2001; 90: 499–504.
- Berin MC, Sampson HA: Mucosal immunology of food allergy. Curr Biol 2013; 23:R389– R400.
- Charbonnier LM, Janssen E, Chou J, Ohsumi TK, Keles S, Hsu JT, et al: Regulatory T-cell deficiency and immune dysregulation, polyendocrinopathy, enteropathy, X-linked-like disorder caused by loss-of-function mutations in LRBA. J Allergy Clin Immunol 2015; 135: 217–227.
- Brough HA, Simpson A, Makinson K, Hankinson J, Brown S, Douiri A, et al: Peanut allergy: effect of environmental peanut exposure in children with filaggrin loss-of-function mutations. J Allergy Clin Immunol 2014; 134: 867–875.e1.
- Li J, Maggadottir SM, Hakonarson H: Are genetic tests informative in predicting food allergy? Curr Opin Allergy Clin Immunol 2016; 16: 257–264.
- Ashley S, Dang T, Koplin J, Martino D, Prescott S: Food for thought: progress in understanding the causes and mechanisms of food allergy. Curr Opin Allergy Clin Immunol 2015; 15: 237–242.
- Scholl I, Untersmayr E, Bakos N, Roth-Walter F, Gleiss A, Boltz-Nitulescu G, et al: Antiulcer drugs promote oral sensitization and hypersensitivity to hazelnut allergens in BALB/c mice and humans. Am J Clin Nutr 2005; 81: 154–160.
- Macia L, Tan J, Vieira AT, Leach K, Stanley D, Luong S, et al: Metabolite-sensing receptors GPR43 and GPR109A facilitate dietary fibre-induced gut homeostasis through regulation of the inflammasome. Nat Commun 2015; 6: 6734.
- de Kivit S, Tobin MC, DeMeo MT, Fox S, Garssen J, Forsyth CB, et al: In vitro evaluation of intestinal epithelial TLR activation in preventing food allergic responses. Clin Immunol 2014; 154: 91–99.
- Stefka AT, Feehley T, Tripathi P, Qiu J, Mc- Coy K, Mazmanian SK, et al: Commensal bacteria protect against food allergen sensitization. Proc Natl Acad Sci USA 2014; 111: 13145–13150.
- Hua X, Goedert JJ, Pu A, Yu G, Shi J: Allergy associations with the adult fecal microbiota: analysis of the American Gut Project. EBio- Medicine 2016; 3: 172–179.
- Berni Canani R, Sangwan N, Stefka AT, Nocerino R, Paparo L, Aitoro R, et al: Lactobacillus rhamnosus GG-supplemented formula expands butyrate-producing bacterial strains in food allergic infants. ISME J 2016; 10: 742–750.
- Wells HG, Osborne TB: The biological reactions of the vegetable protein. I. Anaphylaxis. J Infect Dis 1911; 8: 66–124.
- Hsieh KY, Tsai CC, Wu CH, Lin RH: Epicutaneous exposure to protein antigen and food allergy. Clin Exp Allergy 2003; 33: 1067– 1075.
- Strid J, Hourihane J, Kimber I, Callard R, Strobel S: Epicutaneous exposure to peanut protein prevents oral tolerance and enhances allergic sensitization 1. Clin Exp Allergy 2005; 35: 757–766.
- Tanoue T, Atarashi K, Honda K: Development and maintenance of intestinal regulatory T cells. Nat Rev Immunol 2016; 16: 295– 309.
- Carrier Y, Yuan J, Kuchroo VK, Weiner HL: Th3 cells in peripheral tolerance. I. Induction of Foxp3-positive regulatory T cells by Th3 cells derived from TGF-beta T celltransgenic mice. J Immunol 2007; 178: 179– 185.
- Hadis U, Wahl B, Schulz O, Hardtke-Wolenski M, Schippers A, Wagner N, et al: Intestinal tolerance requires gut homing and expansion of FoxP3+ regulatory T cells in the lamina propria. Immunity 2011; 34: 237–246.
- Cassani B, Villablanca EJ, Quintana FJ, Love PE, Lacy-Hulbert A, Blaner WS, et al: Guttropic T cells that express integrin alpha4beta7 and CCR9 are required for induction of oral immune tolerance in mice. Gastroenterology 2011; 141: 2109–2118.
- Torgerson TR, Linane A, Moes N, Anover S, Mateo V, Rieux-Laucat F, et al: Severe food allergy as a variant of IPEX syndrome caused by a deletion in a noncoding region of the FOXP3 gene. Gastroenterology 2007; 132: 1705–1717.
- Karlsson MR, Rugtveit J, Brandtzaeg P: Allergen- responsive CD4+CD25+ regulatory T cells in children who have outgrown cow’s milk allergy. J Exp Med 2004; 199: 1679–1688.
- Shreffler WG, Wanich N, Moloney M, Nowak-Wegrzyn A, Sampson HA: Association of allergen-specific regulatory T cells with the onset of clinical tolerance to milk protein. J Allergy Clin Immunol 2009; 123: 43–52.
- Jaensson E, Uronen-Hansson H, Pabst O, Eksteen B, Tian J, Coombes JL, et al: Small intestinal CD103+ dendritic cells display unique functional properties that are conserved between mice and humans. J Exp Med 2008; 205: 2139–2149.
- Klebanoff CA, Spencer SP, Torabi-Parizi P, Grainger JR, Roychoudhuri R, Ji Y, et al: Retinoic acid controls the homeostasis of precDC- derived splenic and intestinal dendritic cells. J Exp Med 2013; 210: 1961–1976.
- Coombes JL, Siddiqui KR, Arancibia-Carcamo CV, Hall J, Sun CM, Belkaid Y, et al: A functionally specialized population of mucosal CD103+ DCs induces Foxp3+ regulatory T cells via a TGF-beta and retinoic aciddependent mechanism. J Exp Med 2007; 204: 1757–1764.
- Matteoli G, Mazzini E, Iliev ID, Mileti E, Fallarino F, Puccetti P, et al: Gut CD103+ dendritic cells express indoleamine 2,3-dioxygenase which influences T regulatory/T effector cell balance and oral tolerance induction. Gut 2010; 59: 595–604.
- Szepfalusi Z, Loibichler C, Pichler J, Reisenberger K, Ebner C, Urbanek R: Direct evidence for transplacental allergen transfer. Pediatr Res 2000; 48: 404–407.
- Szepfalusi Z, Loibichler C, Hanel-Dekan S, Dehlink E, Gerstmayr M, Pichler J, et al: Most of diaplacentally transferred allergen is retained in the placenta. Clin Exp Allergy 2006; 36: 1130–1137.
- Macchiaverni P, Ynoue LH, Arslanian C, Verhasselt V, Condino-Neto A: Early exposure to respiratory allergens by placental transfer and breastfeeding. PLoS One 2015; 10:e0139064.
- Abelius MS, Enke U, Varosi F, Hoyer H, Schleussner E, Jenmalm MC, et al: Placental immune response to apple allergen in allergic mothers. J Reprod Immunol 2014; 106: 100–109.
- Abelius MS, Janefjord C, Ernerudh J, Berg G, Matthiesen L, Duchen K, et al: The placental immune milieu is characterized by a Th2- and anti-inflammatory transcription profile, regardless of maternal allergy, and associates with neonatal immunity. Am J Reprod Immunol 2015; 73: 445–459.
- Abelius MS, Lempinen E, Lindblad K, Ernerudh J, Berg G, Matthiesen L, et al: Th2-like chemokine levels are increased in allergic children and influenced by maternal immunity during pregnancy. Pediatr Allergy Immunol 2014; 25: 387–393.
- Netting MJ, Middleton PF, Makrides M: Does maternal diet during pregnancy and lactation affect outcomes in offspring? A systematic review of food-based approaches. Nutrition 2014; 30: 1225–1241.
- Adams J, Voutilainen H, Ullner PM, Jarvinen KM: The safety of maternal elimination diets in breastfeeding mothers with food-allergic infants. Breastfeed Med 2014; 9: 555–556.
- Jarvinen KM, Westfall JE, Seppo MS, James AK, Tsuang AJ, Feustel PJ, et al: Role of maternal elimination diets and human milk IgA in the development of cow’s milk allergy in the infants. Clin Exp Allergy 2014; 44: 69–78.
- Greer FR, Sicherer SH, Burks AW: Effects of early nutritional interventions on the development of atopic disease in infants and children: the role of maternal dietary restriction, breastfeeding, timing of introduction of complementary foods, and hydrolyzed formulas. Pediatrics 2008; 121: 183–191.
- Ierodiakonou D, Garcia-Larsen V, Logan A, Groome A, Cunha S, Chivinge J, et al: Timing of allergenic food introduction to the infant diet and risk of allergic or autoimmune disease: a systematic review and meta-analysis. JAMA 2016; 316: 1181–1192.
- Frazier AL, Camargo CA Jr, Malspeis S, Willett WC, Young MC: Prospective study of peripregnancy consumption of peanuts or tree nuts by mothers and the risk of peanut or tree nut allergy in their offspring. JAMA Pediatr 2014; 168: 156–162.
- Bunyavanich S, Rifas-Shiman SL, Platts- Mills TA, Workman L, Sordillo JE, Camargo CA Jr, et al: Peanut, milk, and wheat intake during pregnancy is associated with reduced allergy and asthma in children. J Allergy Clin Immunol 2014; 133: 1373–1382.
- Lack G, Fox D, Northstone K, Golding J: Factors associated with the development of peanut allergy in childhood. N Engl J Med 2003; 348: 977–985.
- Oyoshi MK, Murphy GF, Geha RS: Filaggrin- deficient mice exhibit TH17-dominated skin inflammation and permissiveness to epicutaneous sensitization with protein antigen. J Allergy Clin Immunol 2009;124:485– 493.e1.
- Du Toit G, Roberts G, Sayre PH, Bahnson HT, Radulovic S, Santos AF, et al: Randomized trial of peanut consumption in infants at risk for peanut allergy. N Engl J Med 2015; 372: 803–813.
- Du Toit G, Sayre PH, Roberts G, Sever ML, Lawson K, Bahnson HT, Brough HA, Santos AF, Harris KM, Radulovic S, Basting M, Turcanu V, Plaut M, Lack G; Immune Tolerance Network LEAP-On Study Team: Effect of avoidance on peanut allergy after early peanut consumption. N Engl J Med 2016;374: 1435–1443.
- Perkin MR, Logan K, Tseng A, Raji B, Ayis S, Peacock J, et al: Randomized trial of introduction of allergenic foods in breast-fed infants. N Engl J Med 2016; 374: 1733–1743.
- Noti M, Kim BS, Siracusa MC, Rak GD, Kubo M, Moghaddam AE, et al: Exposure to food allergens through inflamed skin promotes intestinal food allergy through the thymic stromal lymphopoietin-basophil axis. J Allergy Clin Immunol 2014; 133: 1390– 1399.e1–e6.
- Brown SJ, Asai Y, Cordell HJ, Campbell LE, Zhao Y, Liao H, et al: Loss-of-function variants in the filaggrin gene are a significant risk factor for peanut allergy. J Allergy Clin Immunol 2011; 127: 661–667.
- Sander I, Rihs HP, Doekes G, Quirce S, Krop E, Rozynek P, et al: Component-resolved diagnosis of baker’s allergy based on specific IgE to recombinant wheat flour proteins. J Allergy Clin Immunol 2015; 135: 1529–1537.
- Mandallaz MM, de Weck AL, Dahinden CA: Bird-egg syndrome. Cross-reactivity between bird antigens and egg-yolk livetins in IgE-mediated hypersensitivity. Int Arch Allergy Appl Immunol 1988; 87: 143–150.
- Munblit D, Verhasselt V: Allergy prevention by breastfeeding: possible mechanisms and evidence from human cohorts. Curr Opin Allergy Clin Immunol 2016; 16: 427–433.
- Seppo AE, Autran CA, Bode L, Jarvinen KM: Human milk oligosaccharides and development of cow’s milk allergy in infants. J Allergy Clin Immunol 2017; 139: 708–711.e5.
- Bernard H, Ah-Leung S, Drumare MF, Feraudet- Tarisse C, Verhasselt V, Wal JM, et al: Peanut allergens are rapidly transferred in human breast milk and can prevent sensitization in mice. Allergy 2014; 69: 888–897.
- de Silva D, Geromi M, Halken S, Host A, Panesar SS, Muraro A, et al: Primary prevention of food allergy in children and adults: systematic review. Allergy 2014; 69: 581–589.
- Muraro A, Halken S, Arshad SH, Beyer K, Dubois AE, Du Toit G, et al: EAACI food allergy and anaphylaxis guidelines. Primary prevention of food allergy. Allergy 2014; 69: 590–601.
- di Mauro G, Bernardini R, Barberi S, Capuano A, Correra A, De’ Angelis GL, et al: Prevention of food and airway allergy: consensus of the Italian Society of Preventive and Social Paediatrics, the Italian Society of Paediatric Allergy and Immunology, and Italian Society of Pediatrics. World Allergy Organ J 2016; 9: 28.
- Von Berg A, Koletzko S, Grubl A, Filipiak- Pittroff B, Wichmann HE, Bauer CP, et al: The effect of hydrolyzed cow’s milk formula for allergy prevention in the first year of life: the German Infant Nutritional Intervention Study, a randomized double-blind trial. J Allergy Clin Immunol 2003; 111: 533–540.
- von Berg A, Koletzko S, Filipiak-Pittroff B, Laubereau B, Grubl A, Wichmann HE, et al: Certain hydrolyzed formulas reduce the incidence of atopic dermatitis but not that of asthma: three-year results of the German Infant Nutritional Intervention Study. J Allergy Clin Immunol 2007; 119: 718–725.
- von Berg A, Filipiak-Pittroff B, Kramer U, Link E, Bollrath C, Brockow I, et al: Preventive effect of hydrolyzed infant formulas persists until age 6 years: long-term results from the German Infant Nutritional Intervention Study (GINI). J Allergy Clin Immunol 2008; 121: 1442–1447.
- von Berg A, Filipiak-Pittroff B, Kramer U, Hoffmann B, Link E, Beckmann C, et al: Allergies in high-risk schoolchildren after early intervention with cow’s milk protein hydrolysates: 10-year results from the German Infant Nutritional Intervention (GINI) study. J Allergy Clin Immunol 2013; 131: 1565–1573.
- von Berg A, Filipiak-Pittroff B, Schulz H, Hoffmann U, Link E, Sussmann M, et al: Allergic manifestation 15 years after early intervention with hydrolyzed formulas – the GINI Study. Allergy 2016; 71: 210–219.
- Boyle RJ, Ierodiakonou D, Khan T, Chivinge J, Robinson Z, Geoghegan N, et al: Hydrolysed formula and risk of allergic or autoimmune disease: systematic review and metaanalysis. BMJ 2016; 352:i974.
- Katz Y, Rajuan N, Goldberg MR, Eisenberg E, Heyman E, Cohen A, et al: Early exposure to cow’s milk protein is protective against IgE-mediated cow’s milk protein allergy. J Allergy Clin Immunol 2010; 126: 77–82.
- Du Toit G, Katz Y, Sasieni P, Mesher D, Maleki SJ, Fisher HR, et al: Early consumption of peanuts in infancy is associated with a low prevalence of peanut allergy. J Allergy Clin Immunol 2008; 122: 984–991.
- Togias A, Cooper SF, Acebal ML, Assa’ad A, Baker JR Jr, Beck LA, et al: Addendum guidelines for the prevention of peanut allergy in the United States: summary of the National Institute of Allergy and Infectious Diseasessponsored expert panel. Pediatr Dermatol 2017; 34: 5–12.
- Koplin JJ, Osborne NJ, Wake M, Martin PE, Gurrin LC, Robinson MN, et al: Can early introduction of egg prevent egg allergy in infants? A population-based study. J Allergy Clin Immunol 2010; 126: 807–813.
- Palmer DJ, Metcalfe J, Makrides M, Gold MS, Quinn P, West CE, et al: Early regular egg exposure in infants with eczema: a randomized controlled trial. J Allergy Clin Immunol 2013; 132: 387–392.e1.
- Palmer DJ, Sullivan TR, Gold MS, Prescott SL, Makrides M: Randomized controlled trial of early regular egg intake to prevent egg allergy. J Allergy Clin Immunol 2017;139: 1600–1607.e2.
- Bellach J, Schwarz V, Ahrens B, Trendelenburg V, Aksunger O, Kalb B, et al: Randomized placebo-controlled trial of hen’s egg consumption for primary prevention in infants. J Allergy Clin Immunol 2017;139:1591– 1599.e2.
- Wei-Liang Tan J, Valerio C, Barnes EH, Turner PJ, Van Asperen PA, Kakakios AM, et al: A randomized trial of egg introduction from 4 months of age in infants at risk for egg allergy. J Allergy Clin Immunol 2017;139: 1621–1628.e8.
- Natsume O, Kabashima S, Nakazato J, Yamamoto- Hanada K, Narita M, Kondo M, et al: Two-step egg introduction for prevention of egg allergy in high-risk infants with eczema (PETIT): a randomised, double-blind, placebo-controlled trial. Lancet 2017; 389: 276–286.
- Palmer DJ, Prescott SL: Early introduction of food reduces food allergy – pro. Pediatr Allergy Immunol 2016, Epub ahead of print.
- Szajewska H: Microbiota modulation: can probiotics prevent/treat disease in pediatrics? Nestle Nutr Inst Workshop Ser 2013; 77: 99–110.
- Bunyavanich S, Shen N, Grishin A, Wood R, Burks W, Dawson P, et al: Early-life gut microbiome composition and milk allergy resolution. J Allergy Clin Immunol 2016; 138: 1122–1130.
- Cuello-Garcia CA, Brożek JL, Fiocchi A, et al: Probiotics for the prevention of allergy: a systematic review and meta-analysis of randomized controlled trials. J Allergy Clin Immunol 2015; 136: 952–961.
- Osborn DA, Sinn JK: Prebiotics in infants for prevention of allergy. Cochrane Database Syst Rev 2013;CD006474.
- Boyle RJ, Tang ML, Chiang WC, Chua MC, Ismail I, Nauta A, et al: Prebiotic-supplemented partially hydrolysed cow’s milk formula for the prevention of eczema in highrisk infants: a randomized controlled trial. Allergy 2016; 71: 701–710.
- Dominguez-Bello MG, De Jesus-Laboy KM, Shen N, Cox LM, Amir A, Gonzalez A, et al: Partial restoration of the microbiota of cesarean- born infants via vaginal microbial transfer. Nat Med 2016; 22: 250–253.
- Clemente JC, Dominguez-Bello MG: Safety of vaginal microbial transfer in infants delivered by caesarean, and expected health outcomes. BMJ 2016; 352:i1707.
- Simpson EL, Chalmers JR, Hanifin JM, Thomas KS, Cork MJ, McLean WH, et al: Emollient enhancement of the skin barrier from birth offers effective atopic dermatitis prevention. J Allergy Clin Immunol 2014; 134: 818–823.
- Horimukai K, Morita K, Narita M, Kondo M, Kitazawa H, Nozaki M, et al: Application of moisturizer to neonates prevents development of atopic dermatitis. J Allergy Clin Immunol 2014; 134: 824–830.e6.
- Kelleher M, Dunn-Galvin A, Hourihane JO, Murray D, Campbell LE, McLean WH, et al: Skin barrier dysfunction measured by transepidermal water loss at 2 days and 2 months predates and predicts atopic dermatitis at 1 year. J Allergy Clin Immunol 2015; 135: 930– 935.e1.
- Kelleher MM, Dunn-Galvin A, Gray C, Murray DM, Kiely M, Kenny L, et al: Skin barrier impairment at birth predicts food allergy at 2 years of age. J Allergy Clin Immunol 2016; 137: 1111–1116.e1–e8.
- Berni Canani R, Nocerino R, Terrin G, Frediani T, Lucarelli S, Cosenza L, et al: Formula selection for management of children with cow’s milk allergy influences the rate of acquisition of tolerance: a prospective multicenter study. J Pediatr 2013; 163: 771– 777.e1.
- Berni Canani R, Di Costanzo M, Bedogni G, Amoroso A, Cosenza L, Di Scala C, et al: Extensively hydrolysed casein formula containing Lactobacillus rhamnosus GG reduces the occurrence of other allergic manifestations in children with cow’s milk allergy: 3-year randomized controlled trial. J Allergy Clin Immunol 2016, Epub ahead of print.
- Gernez Y, Nowak-Węgrzyn A: Immunotherapy for food allergy: are we there yet? J Allergy Clin Immunol Pract 2017;5:250–272.
- Vickery BP, Berglund JP, Burk CM, Fine JP, Kim EH, Kim JI, et al: Early oral immunotherapy in peanut-allergic preschool children is safe and highly effective. J Allergy Clin Immunol 2017; 139: 173–181.e8.
- Wood RA, Kim JS, Lindblad R, Nadeau K, Henning AK, Dawson P, et al: A randomized, double-blind, placebo-controlled study of omalizumab combined with oral immunotherapy for the treatment of cow’s milk allergy. J Allergy Clin Immunol 2016; 137: 1103–1110.e1–e11.
- MacGinnitie AJ, Rachid R, Gragg H, Little SV, Lakin P, Cianferoni A, et al: Omalizumab facilitates rapid oral desensitization for peanut allergy. J Allergy Clin Immunol 2017;139: 873–881.e8.
- Chen Y, Kuchroo VK, Inobe J, Hafler DA, Weiner HL: Regulatory T cell clones induced by oral tolerance: suppression of autoimmune encephalomyelitis. Science 1994; 265: 1237–1240.
- Fukaura H, Kent SC, Pietrusewicz MJ, Khoury SJ, Weiner HL, Hafler DA: Induction of circulating myelin basic protein and proteolipid protein-specific transforming growth factor-beta1-secreting Th3 T cells by oral administration of myelin in multiple sclerosis patients. J Clin Invest 1996; 98: 70–77.
- Escudero C, Rodriguez Del Rio P, Sanchez- Garcia S, Perez-Rangel I, Perez-Farinos N, Garcia-Fernandez C, et al: Early sustained unresponsiveness after short-course egg oral immunotherapy: a randomized controlled study in egg-allergic children. Clin Exp Allergy 2015; 45: 1833–1843.
- Pajno GB, Caminiti L, Ruggeri P, De Luca R, Vita D, La Rosa M, et al: Oral immunotherapy for cow’s milk allergy with a weekly updosing regimen: a randomized single-blind controlled study. Ann Allergy Asthma Immunol 2010; 105: 376–381.
- Nowak-Wegrzyn A, Szajewska H, Lack G: Food allergy and the gut. Nat Rev Gastroenterol Hepatol 2017;14:241–257.